Genetic Engineering: Key Principles, Methods, and Groundbreaking Applications in Modern Science
Genetic engineering is revolutionizing science and technology with its ability to modify DNA and create enhanced organisms. This article delves into the core principles of genetic engineering, its history, methods, and the wide range of applications that span from agriculture and medicine to environmental conservation and biotechnology. It also discusses the ethical concerns, risks, and future potential of this transformative field.
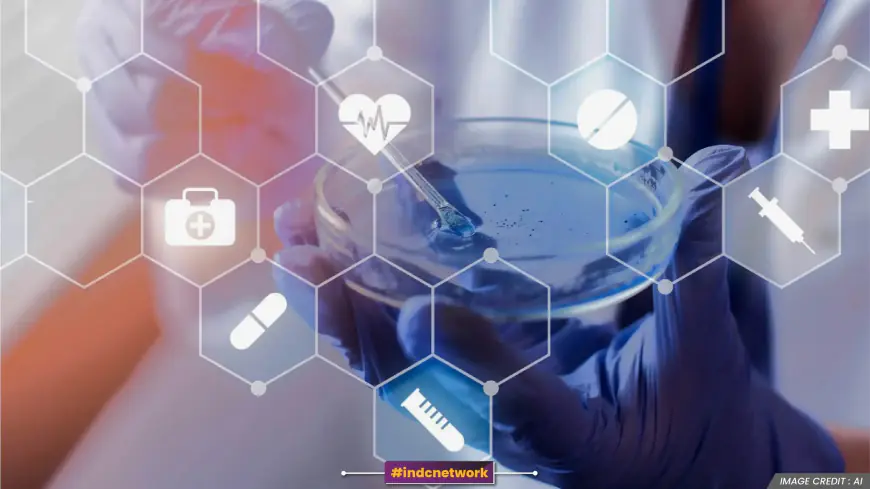
INDC Network : Science : Genetic Engineering: Key Principles, Methods, and Groundbreaking Applications in Modern Science
Introduction to Genetic Engineering : Genetic engineering, often referred to as genetic modification, is a field that involves the direct manipulation of an organism’s DNA to alter its characteristics in a precise and controlled manner. It allows scientists to insert, delete, or modify specific genes, opening the door to numerous possibilities in medicine, agriculture, and other fields. With advancements in technology, particularly CRISPR-Cas9, genetic engineering has become more accessible and powerful, raising the potential for groundbreaking innovations while simultaneously invoking ethical debates.
The technology’s roots trace back to the discovery of DNA's structure by James Watson and Francis Crick in 1953, followed by the development of molecular biology techniques in the 1970s. Today, genetic engineering is integral to fields like biotechnology, healthcare, and environmental science, and is likely to reshape the future of these sectors.
Principles of Genetic Engineering
Genetic engineering relies on several core principles and methodologies, which provide the foundation for its wide-ranging applications.
1. Gene Identification and Isolation : The first step in genetic engineering involves identifying the gene responsible for a specific trait. Scientists must locate the precise DNA sequence that needs alteration or introduction. This process often uses tools such as DNA sequencing and bioinformatics to map and analyze genes.
Once the target gene is identified, it must be isolated from the DNA strand. Enzymes, especially restriction endonucleases, are commonly employed for this task. These enzymes act as molecular scissors, cutting DNA at specific sites to extract the desired gene.
2. Gene Insertion : The next step is inserting the isolated gene into a host organism. This is accomplished by using vectors—vehicles that transport genetic material into cells. Common vectors include plasmids (circular DNA molecules found in bacteria) and viral vectors, which leverage a virus's ability to introduce DNA into host cells.
Once inside, the gene integrates into the host's genome, either replacing an existing gene or adding a new one. The insertion must be carefully managed to avoid unintended side effects, such as disrupting other essential genes.
3. Gene Expression : After the gene is inserted, it must be expressed in the host organism. This involves turning the gene "on" so that it produces the desired protein or trait. Scientists monitor and regulate gene expression using promoters, enhancers, and other regulatory sequences.
4. Screening and Selection : The final principle is ensuring that the modification has been successful. This involves screening the host organism to verify that the inserted gene is functioning correctly and producing the desired results. Selection markers, such as antibiotic resistance genes, are sometimes used to identify successfully modified organisms.
Methods of Genetic Engineering
Several techniques are utilized in genetic engineering, each with its own advantages and specific use cases. The following methods are among the most widely employed in modern genetic research.
1. Recombinant DNA Technology : Recombinant DNA (rDNA) technology involves combining DNA from two or more sources into a single molecule. This is one of the most fundamental methods in genetic engineering, used to create genetically modified organisms (GMOs). The process typically involves:
- Cutting the target gene from the donor organism using restriction enzymes.
- Inserting the gene into a plasmid vector.
- Transforming the host organism (usually bacteria) with the recombinant plasmid.
- Multiplying the host cells to produce large quantities of the recombinant protein.
2. CRISPR-Cas9 : Clustered Regularly Interspaced Short Palindromic Repeats (CRISPR) combined with the Cas9 enzyme has revolutionized genetic engineering. This method allows for precise, targeted modifications to an organism’s DNA by using a guide RNA to direct the Cas9 enzyme to the specific site on the genome.
The CRISPR-Cas9 system has several advantages:
- Precision: CRISPR can target specific locations in the genome with incredible accuracy.
- Efficiency: The method is faster and more cost-effective compared to traditional genetic engineering techniques.
- Versatility: CRISPR can be used in a variety of organisms, including plants, animals, and humans.
3. Gene Silencing and RNA Interference (RNAi) : Gene silencing techniques like RNA interference (RNAi) inhibit the expression of specific genes. RNAi uses small RNA molecules (siRNA or shRNA) to target mRNA transcripts, preventing translation into proteins. This is useful in studying gene function and creating knockdown organisms.
4. Somatic Cell Nuclear Transfer (SCNT) : SCNT is a method of cloning that involves transferring the nucleus of a somatic cell (non-reproductive) into an enucleated egg cell. This technique was famously used to clone Dolly the sheep in 1996 and remains a vital tool in cloning research and regenerative medicine.
Applications of Genetic Engineering
Genetic engineering has found applications across a wide range of fields, from agriculture and healthcare to environmental management and industrial biotechnology.
1. Agricultural Biotechnology : One of the most significant areas impacted by genetic engineering is agriculture. Genetically modified crops have been developed to increase yields, resist pests and diseases, and tolerate harsh environmental conditions. Examples include:
- Bt Crops: These crops contain genes from the bacterium Bacillus thuringiensis, which produces a protein toxic to specific insect pests.
- Herbicide-Resistant Crops: Plants like soybeans and corn have been engineered to withstand herbicides, allowing farmers to control weeds without damaging crops.
GM crops not only improve food security but also reduce the need for chemical pesticides and herbicides, contributing to sustainable farming practices.
2. Medicine and Gene Therapy : In medicine, genetic engineering offers the potential for treating genetic disorders through gene therapy. Gene therapy involves correcting defective genes responsible for disease development. By inserting healthy genes into the cells of patients with inherited conditions like cystic fibrosis or sickle cell anemia, scientists aim to cure these diseases at the genetic level. CRISPR-based therapies are currently being explored for their potential to treat cancer, HIV, and a range of inherited disorders. The first human trials using CRISPR have shown promising results, bringing the dream of personalized medicine closer to reality.
3. Environmental Conservation : Genetic engineering is playing a growing role in environmental conservation efforts. Scientists are exploring the potential for genetically modifying organisms to mitigate environmental issues. For example:
- Bio-remediation: Bacteria can be engineered to break down pollutants, such as oil spills or plastic waste, in the environment.
- Conservation of endangered species: Genetic techniques are being used to help preserve and even de-extinct species, such as through cloning or genetic rescue of endangered populations.
4. Industrial Biotechnology : In industrial settings, genetically engineered microorganisms are used to produce biofuels, pharmaceuticals, and biodegradable materials. These organisms can be designed to carry out specific biochemical processes, offering sustainable alternatives to traditional manufacturing.
5. Synthetic Biology : Synthetic biology is an emerging field that combines genetic engineering with systems biology and engineering principles. By designing and building new biological parts, systems, or entire organisms, synthetic biology aims to create novel solutions for challenges in medicine, agriculture, and energy production.
Ethical Concerns and Challenges
Despite its many benefits, genetic engineering raises significant ethical concerns. The ability to alter life at a fundamental level leads to debates about the morality of such actions, especially when it comes to human genetic modification. Some of the key ethical issues include:
1. Designer Babies : The prospect of using genetic engineering to create "designer babies" by selecting traits such as intelligence, appearance, or athletic ability is highly controversial. Critics argue that this could lead to a new form of eugenics, deepening social inequality.
2. Germline Modifications : Germline editing involves altering the DNA in reproductive cells, meaning the changes will be passed down to future generations. This raises concerns about unforeseen consequences and the long-term impacts on the human gene pool.
3. Environmental Risks : The release of genetically modified organisms (GMOs) into the environment may have unintended ecological consequences. For instance, genetically modified crops could potentially crossbreed with wild relatives, leading to the spread of modified genes in natural ecosystems.
4. Intellectual Property : The patenting of genetically engineered organisms and related technologies raises questions about ownership and access. Critics argue that large biotech companies hold too much power over essential agricultural and medical technologies.
Future Potential of Genetic Engineering
The future of genetic engineering is both exciting and uncertain. Advances in CRISPR and other gene-editing tools promise to unlock new possibilities in treating diseases, enhancing food production, and even combating climate change. However, the field will need to navigate complex ethical and regulatory challenges to ensure that the benefits of genetic engineering are realized responsibly and equitably.
Potential future applications include:
- Gene editing for disease resistance: Creating plants and animals that are resistant to diseases without the need for chemicals or vaccines.
- Enhanced humans: Beyond treating genetic disorders, genetic engineering may one day be used to enhance human abilities, such